Virogenesis: The Story of Life’s Not-So-Distant Cousin
What are viruses, how do they work, and where did they come from?
Over the last 3.5 billion years or so, our planet has been home to the most bizarre phenomenon in the known universe: life. Across continents and seas, planet Earth is filled with organic, self-replicating machines ranging from simple bacteria to the humans reading this article. For over 200 years since the discovery of microbial life, it was thought that the world of biochemistry ended with unicellular organisms. However, in 1892, our understanding of the living world would change forever.
In 1892, Russian microbiologist Dmitry Ivanovsky would uncover the elusive source of tobacco mosaic disease – an infection contracted by tobacco plants. Like his contemporaries, Ivanovsky hypothesized that the disease was not caused by a bacterium, as no such organisms had been detected. Rather, he discovered a smaller, stranger pathogen: the virus.
Named after the Latin word for “poisonous substance,” viruses are the nextdoor neighbors to life on Earth. Somewhere in a grey area between living and nonliving, viruses have fascinated, perplexed, and terrified biologists for almost 129 years. They are one of our greatest adversaries, wreaking havoc ranging from a simple cough to the current pandemic, which has claimed over 4.5 million lives thus far. In researching viruses for over a century, the scientific community has learned a great deal about what they are, how they work, and what they are made of.
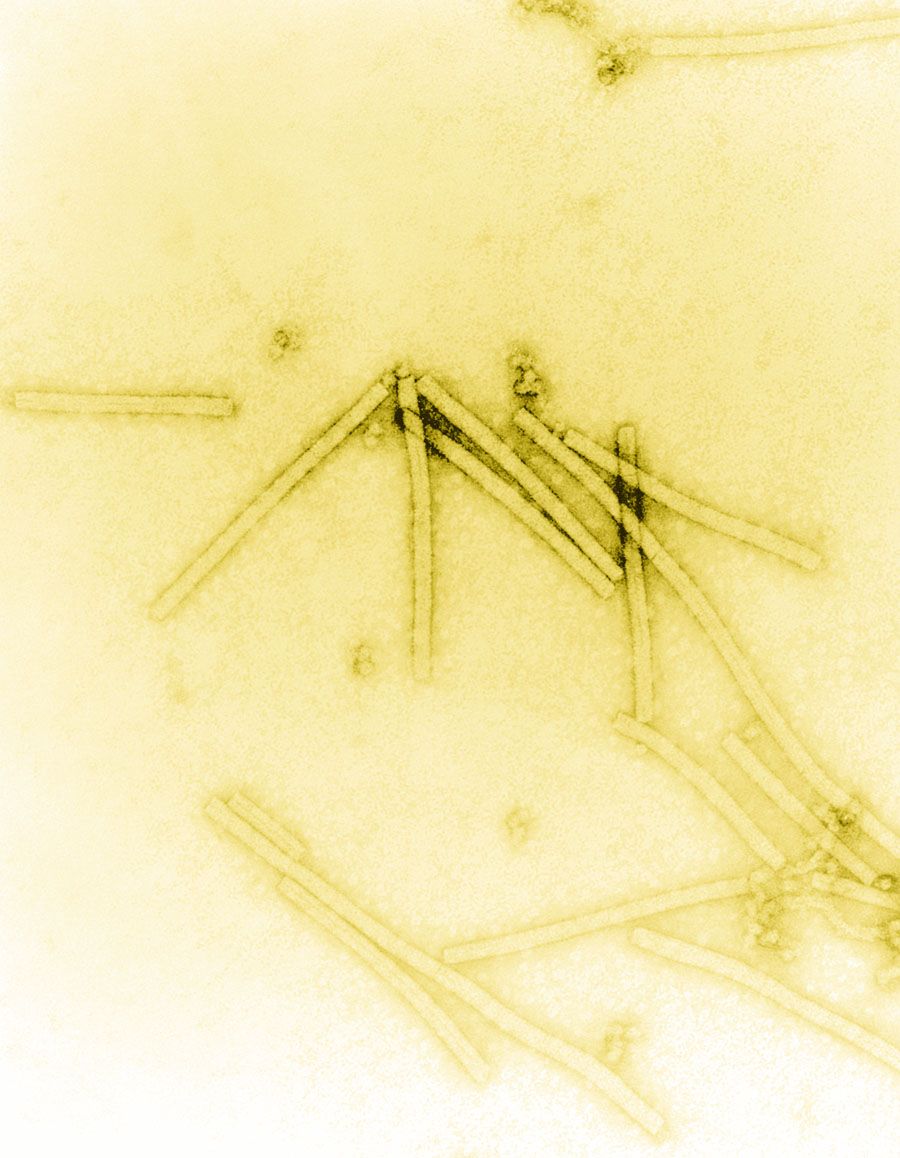
Though diverse in size, shape, and structure, all viruses can be boiled down to three major components: a protein-based shell called a capsid, a DNA or RNA sequence – which can be either single-stranded or double-stranded – and an outer envelope made from lipids and proteins. Not all viruses have envelopes, strangely enough, and this is something important to discuss later. For now, we will analyze viruses based on their commonalities.
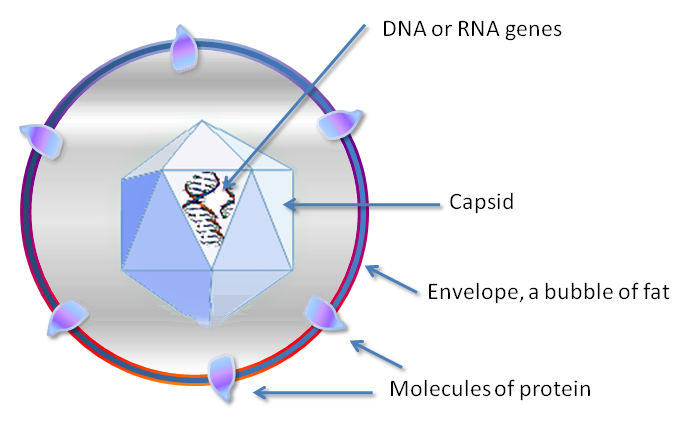
Viruses are, as stated before, not exactly alive, and not exactly nonliving. They are a sort of intermediate, and this is precisely why they are so unique. Though many viruses can perform different functions – another topic we will return to soon – all viruses are characterized by one common characteristic: an inability to reproduce on their own.
Viruses, like all parasites, are dependent on host organisms to carry out their basic functions. However, while some parasites may use host organisms to contain eggs or as food for their offspring, viruses quite literally depend on host cells to replicate. Along a given virus are a set of receptor proteins that are scattered throughout the capsid and envelope. These receptors are specialized in nature, fine-tuned over billions of years to bind to membrane proteins carried by a given host. Once they have attached themselves to a host cell, the shape of their receptors changes along with the proteins they have bound to. At this stage, one of two things will happen, depending on the type of virus.
For some viruses, the next stage is to use the proteins they have bound to to break open their capsid, injecting their genetic material into the host cell. Other viruses, however, enter the cell fully intact, using the membrane proteins around them to facilitate endocytosis – that is, the cell membrane folds and forms a transport vesicle containing the virus. Viruses that do this subsequently release their genetic material into the cell.
Whatever a virus does in order to enter a cell, the next step is perhaps the most gruesome. As their genetic material approaches cellular machines such as ribosomes and RNA polymerases, the genetic material of the virus is used by the cell to replicate viral components such as capsid proteins and parts of the viral envelope. Depending on whether or not a virus uses DNA or RNA, it may use a variety of enzymes to replicate the genetic material, such as reverse transcriptases – enzymes that produce complementary DNA from genomic RNA. Alternatively, it may just use standard DNA polymerases to replicate DNA, single-stranded or double-stranded. Once the cell is completely filled with viral copies, the viruses will use a variety of proteins – such as endolysin – to break open the cell membrane, killing the cell and releasing the viral horde into the surrounding environment. The process will repeat a countless number of times, spreading from cell to cell unless stopped by antibodies or other means, such as oseltamivir, a drug used to treat influenza.
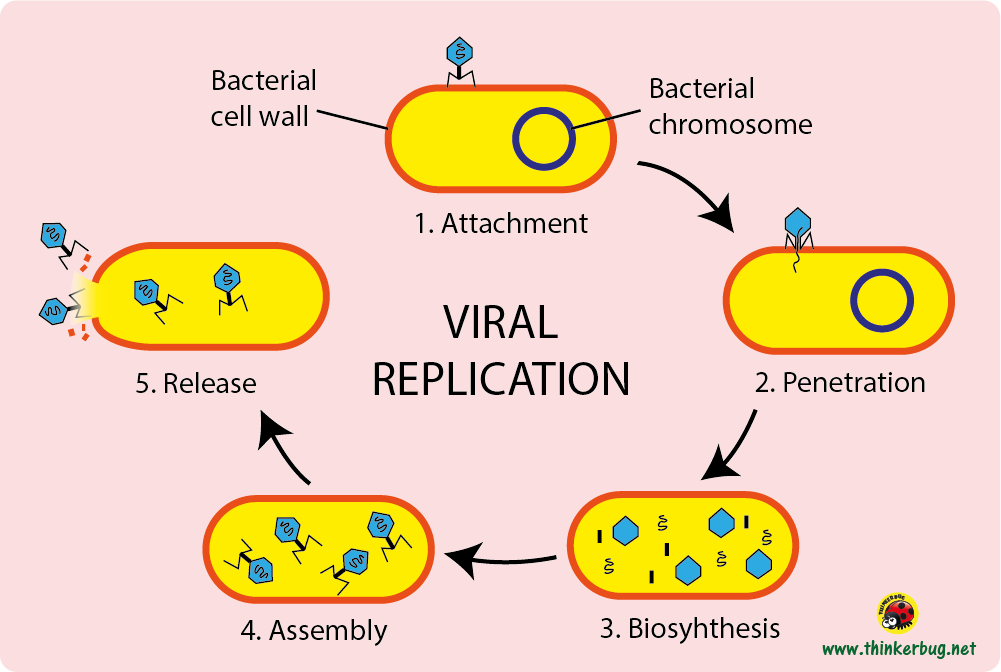
Because viruses use cellular machinery to reproduce, one would think that their origin is the same as the cells they infect – that is, that viruses evolved from a common ancestor. Even if there was such a thing, though, the question still remains: where did the first virus come from? The answer is – like all things – complicated.
As explained before, viruses come in a wide variety of shapes and forms. Some use DNA as their genetic code, which may be single-stranded or double-stranded – while others use RNA in either form. Some viruses have small, simple capsids that get the job done without any extra features. Others, however, possess envelopes with a similar layout to a cell membrane, with proteins and lipids scattered throughout. How, then, could all viruses be the same?
As our understanding of viruses has progressed, a set of three major theories has arisen to try and explain where they came from. The first in question is known as the “progressive hypothesis.” According to this theory, viruses are – in a sense – defective components of life itself. In living cells, pieces of genetic material are capable of moving throughout the genome. These fragments – named “mobile genetic elements” – are said to have developed over time so that they may have transferred from cell to cell, perhaps in a similar fashion to bacterial conjugation – where the genetic information of two bacterial cells is recombined and redistributed between the two.
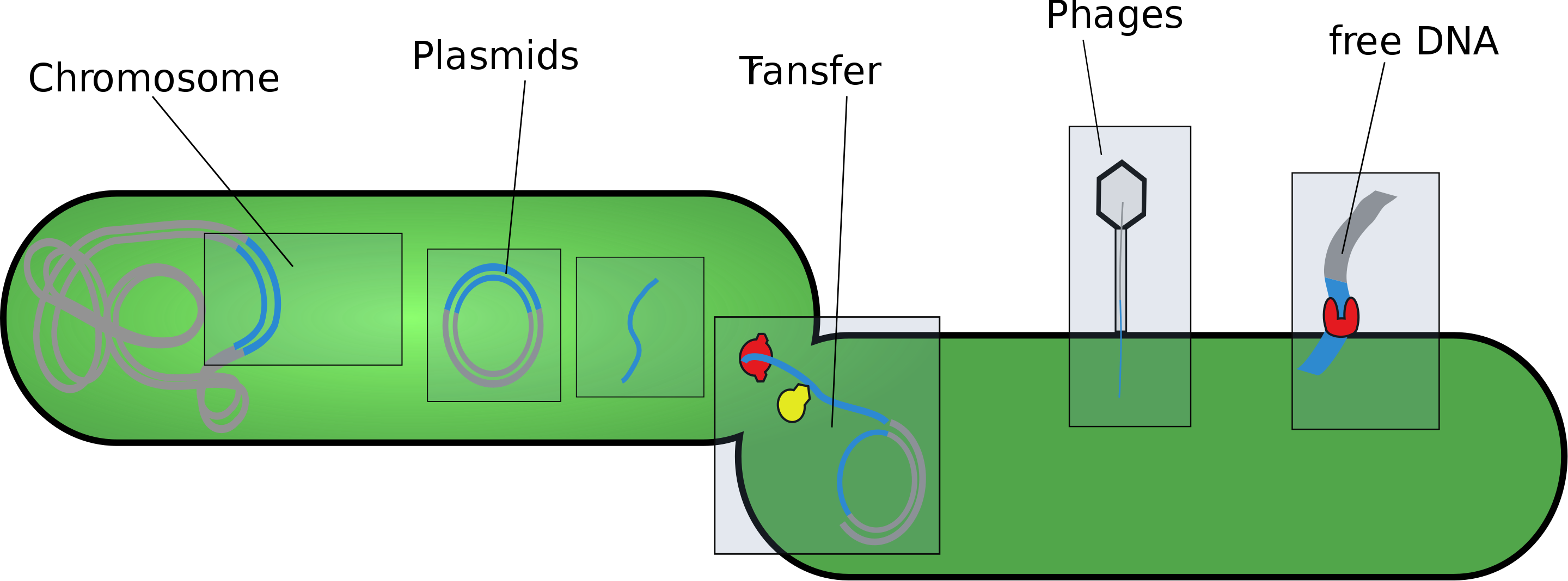
An example of this hypothesis can be seen in retroviruses – a group of viruses that contain single-stranded RNA and use reverse transcriptases to produce the DNA that is ultimately transcribed into new copies of the viral genome. If retroviral RNA originally existed as mobile genetic elements, then it certainly makes sense that they would reproduce this way. Perhaps mobile RNA elements passed from cell to cell were developed and mutated over time so that they encoded the capsid proteins that make up viruses such as HIV and HTLV-1. This process could, in theory, follow the same process using single-stranded or double-stranded DNA.
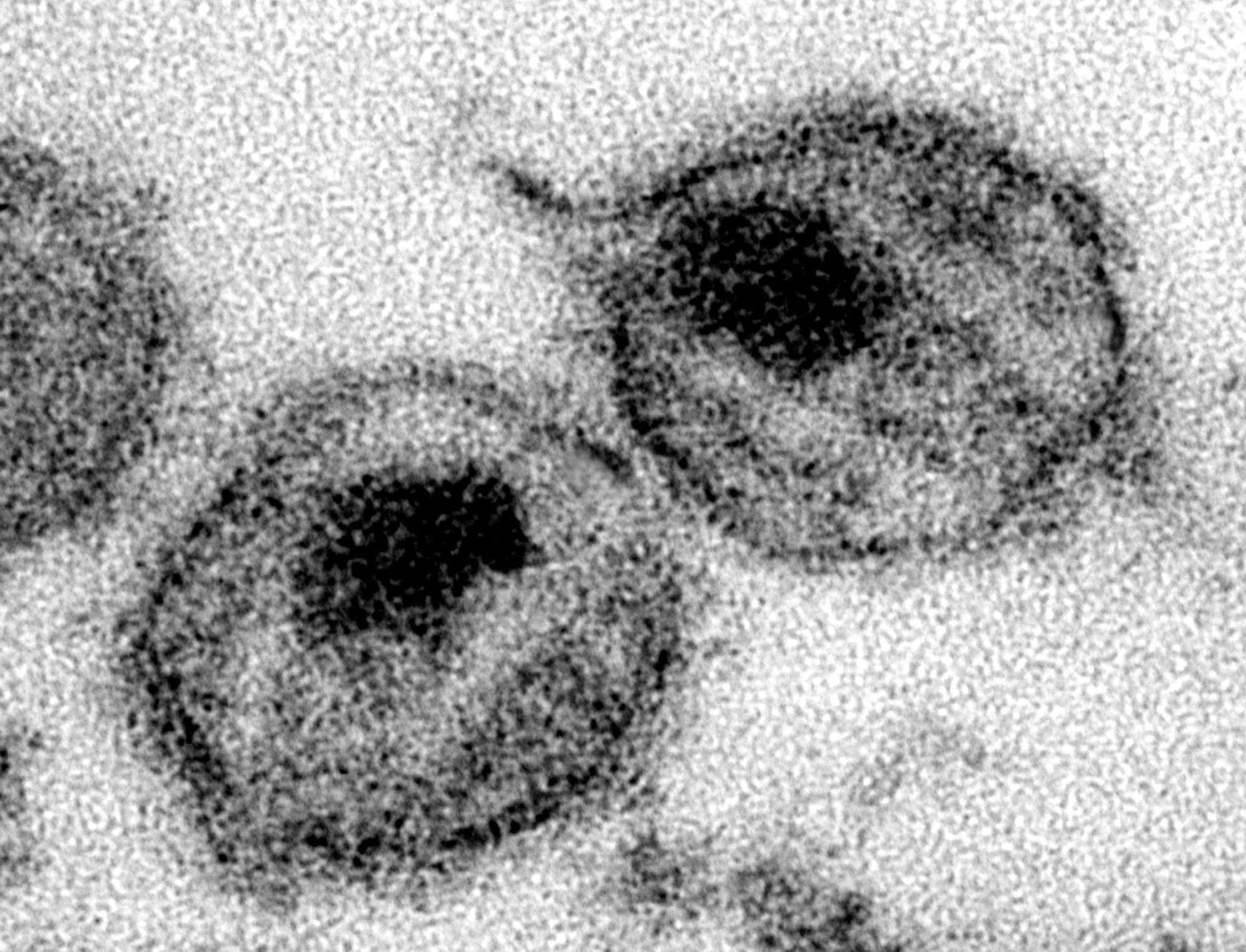
The second hypothesis is known as the “regressive hypothesis.” According to this theory, parasitic microbes like Candidatus Rickettsia andeanae – a cause of spotted fever – may have grown so dependent on their host organisms that they ultimately gave up the need for autonomy. Using their host as a means of self-replication, they would – according to the regressive hypothesis – no longer have the need to possess the full genome of a living cell, instead letting their genetic code deteriorate until it encoded a sparse set of proteins and lipids. To see this idea in real life, we must look to the viral phylum Nucleocytoviricota.
Nucleocytoviricota contains some of the largest known viruses, reaching sizes upwards of 350 nanometers. They are noted to possess massive viral envelopes and a wide variety of internal and external proteins, many of which make up their intricate capsids. What surprised virologists most of all, though, was the discovery that some of the genes encoded into members of the phylum Nucleocytoviricota performed complex internal reactions, similar to those found in living cells. Perhaps – over millions of years – the ancestors to Nucleocytoviricota arose from parasitic microbes that underwent the process laid out by the regressive hypothesis.
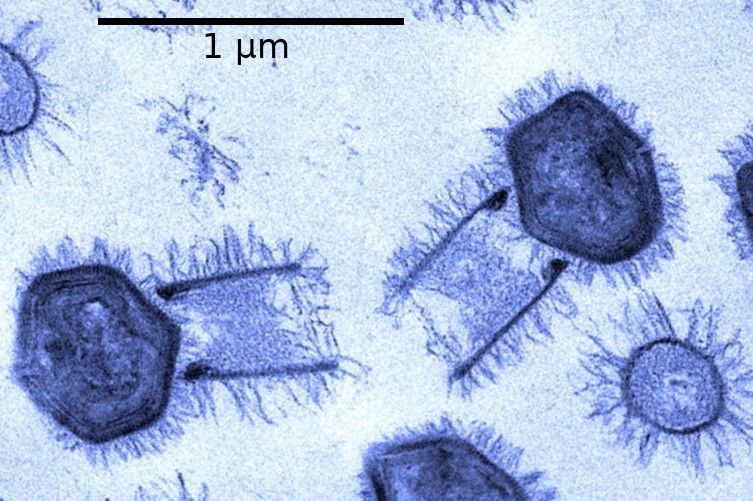
The last hypothesis – and perhaps the most controversial – is the “virus first” hypothesis. In this theory, viruses such as Nucleocytoviricota are a sort of proto-organism – that is, they are the precursors to life on Earth. In a way, this makes sense as well. A wide variety of viruses – whatever genetic code they may use – possess genes that don’t necessarily have cellular analogues, indicating evolutionary independence from living organisms. The hypothesis suggests that viruses such as those with envelopes were the precursor to the first cell – a sort of “protocell,” if you will. Slowly but surely, these protocells may have developed fully-fledged lipid membranes instead of envelopes, mutating further to engage in metabolic reactions.
Truly, viruses are a perplexing, fascinating companion to life on Earth, and in due time we may hopefully understand them to a greater degree.